Bcs Class 4
The Biopharmaceutics Classification System is a system to differentiate the drugs on the basis of their solubility and permeability.[1]
Jump to BCS classes - According to the Biopharmaceutical Classification System (BCS) drug substances are classified to four classes upon their solubility. Jump to Conclusion. Fabricated aprepitant particles of size 300 mm but it was not proved successful as a pretreatment process for ibuprofen in the present case.
This system restricts the prediction using the parameters solubility and intestinal permeability. The solubility classification is based on a United States Pharmacopoeia (USP) aperture. The intestinal permeability classification is based on a comparison to the intravenous injection. All those factors are highly important because 85% of the most sold drugs in the United States and Europe are orally administered[citation needed].
BCS classes[edit]
According to the Biopharmaceutical Classification System (BCS) drug substances are classified to four classes upon their solubility and permeability:[1]
- Class I - high permeability, high solubility
- Example: metoprolol, paracetamol[2]
- Those compounds are well absorbed and their absorption rate is usually higher than excretion.
- Class II - high permeability, low solubility
- Example: glibenclamide, bicalutamide, ezetimibe, aceclofenac
- The bioavailability of those products is limited by their solvation rate. A correlation between the in vivo bioavailability and the in vitro solvation can be found.
- Class III - low permeability, high solubility
- Example: cimetidine
- The absorption is limited by the permeation rate but the drug is solvated very fast. If the formulation does not change the permeability or gastro-intestinal duration time, then class I criteria can be applied.
- Class IV - low permeability, low solubility
- Example: Bifonazole
- Those compounds have a poor bioavailability. Usually they are not well absorbed over the intestinal mucosa and a high variability is expected.
Definitions[edit]
The drugs are classified in BCS on the basis of solubility, permeability, and dissolution.
Solubility class boundaries are based on the highest dose strength of an immediate release product. A drug is considered highly soluble when the highest dose strength is soluble in 250 ml or less of aqueous media over the pH range of 1 to 7.5. The volume estimate of 250 ml is derived from typical bioequivalence study protocols that prescribe administration of a drug product to fasting human volunteers with a glass of water.
Permeability class boundaries are based indirectly on the extent of absorption of a drug substance in humans and directly on the measurement of rates of mass transfer across human intestinal membrane. Alternatively non-human systems capable of predicting drug absorption in humans can be used (such as in-vitro culture methods). A drug substance is considered highly permeable when the extent of absorption in humans is determined to be 90% or more of the administered dose based on a mass-balance determination or in comparison to an intravenous dose.
For dissolution class boundaries, an immediate release product is considered rapidly dissolving when no less than 85% of the labeled amount of the drug substance dissolves within 15 minutes using USP Dissolution Apparatus 1 at 100 RPM or Apparatus 2 at 50 RPM in a volume of 900 ml or less in the following media: 0.1 N HCl or simulated gastric fluid or pH 4.5 buffer and pH 6.8 buffer or simulated intestinal fluid.
See also[edit]
- ADME
References[edit]
- ^ abMehta M (2016). Biopharmaceutics Classification System (BCS): Development, Implementation, and Growth. Wiley. ISBN978-1-118-47661-1.
- ^https://www.ema.europa.eu/documents/scientific-guideline/draft-paracetamol-oral-use-immediate-release-formulations-product-specific-bioequivalence-guidance_en.pdf
Further reading[edit]
- Folkers G, van de Waterbeemd H, Lennernäs H, Artursson P, Mannhold R, Kubinyi H (2003). Drug Bioavailability: Estimation of Solubility, Permeability, Absorption and Bioavailability (Methods and Principles in Medicinal Chemistry). Weinheim: Wiley-VCH. ISBN3-527-30438-X.
- Amidon GL, Lennernäs H, Shah VP, Crison JR (March 1995). 'A theoretical basis for a biopharmaceutic drug classification: the correlation of in vitro drug product dissolution and in vivo bioavailability'. Pharm. Res. 12 (3): 413–20. PMID7617530.
External links[edit]
- BCS guidance of the U.S. Food and Drug Administration
Drug nanocrystals are known to increase the solubility of Biopharmaceutics Classification System (BCS) class II and IV drugs. SmartCrystals are the second generation nanocrystals with particle size of less than 100 nm and increased the stability and solubility of drug and drug product. The combinative methods adopted for the preparation of SmartCrystals are reported to shorten the processing time to reduce the particle size of the drug. This study was carried out with the aim to prepare nanosuspensions of aprepitant and ibuprofen using two pretreatment methods, precipitation and ball milling in a combination of high-pressure homogenisation (HPH). Ball milling and precipitation resulted in nanosuspensions having a particle size less than 1 µ, which were subjected to high HPH. HPH further led to a reduction in the particle size. However, the precipitation method failed to reduce the size of ibuprofen particles to 1 µ.
1. Introduction
Water insolubility is one of the central elements which restrict the usage of many potential drug moieties and other active compounds. Because of this, the bioavailability of the drug is less and it fails to reach the site of action. Drug nanocrystal is a widely used approach, invented in 1990, to increase the solubility of drugs and other active compounds. The method of preparation of nanocrystals is broadly classified as top-down and bottom-up. Top-down method comprises high-pressure homogenisation (HPH) and ball milling whereas spray drying and precipitation come under the bottom-up approaches.[1Muller RH, Gohla S, Keck CM. State of the art of nanocrystals – special features, production, nanotoxicology aspects and intracellular delivery. Eur J Pharm Biopharm. 2011;78:1–9.
Junghanns J-UAH, Muller RH. Nanocrystal technology, drug delivery and clinical applications. Int J Nanomed. 2008;3:295–309.–3Merisko-Liversidge E, Liversidge GG. Nanosizing for oral and parenteral drug delivery: a perspective on formulating poorly-water soluble compounds using wet media milling technology. Adv Drug Deliv Rev. 2011;63:427–440.[Crossref], [PubMed], [Web of Science ®], [Google Scholar]] SmartCrystals are the second generation nanocrystals, having particle size less than 100 nm and possess few advantages, such as quicker production, improved physical stability and improved in vivo performances. SmartCrystal is the term used for the nanocrystal or nanosuspension prepared by combinations of the top-down and bottom-up methods, such as precipitation, ball milling, lyophilisation or spray drying along with HPH.[4Keck C, Kobierski S, Mauludin R, et al. Second generation of drug nanocrystals for delivery of poorly soluble drugs: Smartcrystal technology. Dosis. 2008;24:124–128.[Google Scholar],5Shegokar R, Muller RH. Nanocrystals: industrially feasible multifunctional formulation technology for poorly soluble actives. Int J Pharm. 2010;399:129–139.[Crossref], [PubMed], [Web of Science ®], [Google Scholar]] The SmartCrystal technology is owned by Abott and employs combinations of the above-mentioned methods to prepare nanocrystals.[3Merisko-Liversidge E, Liversidge GG. Nanosizing for oral and parenteral drug delivery: a perspective on formulating poorly-water soluble compounds using wet media milling technology. Adv Drug Deliv Rev. 2011;63:427–440.[Crossref], [PubMed], [Web of Science ®], [Google Scholar]]
In our laboratory setup, we have made an attempt to prepare SmartCrystals (nanosuspension) of aprepitant and ibuprofen by various combinations of methods. Aprepitant is an antiemetic, used for prevention of both the acute and delayed cancer chemotherapy-induced nausea and vomiting. It mediates its effect by the antagonism of neurikinin-1 (NK-1) receptors located in the brainstem nuclei of the dorsal vagal complex. Aprepitant is chosen as a model drug as it is a Biopharmaceutics Classification System (BCS) class IV drug, having low solubility and low permeability.[6Chawla SP, Grunberg SM, Gralla RJ, et al. Establishing the dose of the oral NK1 antagonist aprepitant for the prevention of chemotherapy-induced nausea and vomiting. Cancer. 2003;97:2290–2300.[Crossref], [PubMed], [Web of Science ®], [Google Scholar]] Ibuprofen is one of the widely used analgesic and anti-inflammatory agents. It is a BCS class II drug, having a high permeability but low solubility.[7Kockbek P, Baumgartner S, Kristl J. Preparation and evaluation of nanosuspensions for enhancing the dissolution of poorly soluble drugs. Int J Pharm. 2006;312:179–186.[Crossref], [PubMed], [Web of Science ®], [Google Scholar]] This study was carried out with the aim to prepare nanosuspensions of aprepitant and ibuprofen by second generation techniques.
2. Methods
2.1. Preparation of nanosuspensions
Two combination methodologies viz. the H69 process, combination of precipitation followed by HPH and combinative technologies (CT), combination of ball milling accompanied by HPH, were tried to prepare nanosuspensions (SmartCrystals) of two drugs, aprepitant and ibuprofen.[3Merisko-Liversidge E, Liversidge GG. Nanosizing for oral and parenteral drug delivery: a perspective on formulating poorly-water soluble compounds using wet media milling technology. Adv Drug Deliv Rev. 2011;63:427–440.[Crossref], [PubMed], [Web of Science ®], [Google Scholar],8–10Moschwitzer JP, Lemke A. Gentle method for preparing ultrafine particle suspensions, useful for formulating e.g. pharmaceuticals, cosmetics, foods or pesticides, comprises freezing non-aqueous solution then dispersion of the frozen product. German patent application DE102005053462 A1. 2007 May 10.
Kobierski S, Keck CM. Nanocrystal production by BMHPH combination technology. Controlled Release Society, Abstract, Germany Chapter, Annual Meeting, 2008 Mar 4–5, 40.
Peterson R. Nanocrystals for use in topical cosmetic formulations and method of production thereof. U.S. patent 0,047,297 A1. 2010Feb 25.] Precipitation and ball milling were termed as pretreatment methods. Different stabilisers (surfactant or polymeric), such as sodium lauryl sulfate (SLS), tween 80, polyvinyl alcohol (PVA), poloxamer 188 and poloxamer 407 were used per se and in combinations. The formulation batches of aprepitant and ibuprofen were given codes (enlisted in Tables 1 and 2) depending on the concentration of drug, stabilisers and pretreatment methods.
Published online:
17 June 2015Table 1. Formulation batches by the CT process (ball milling + HPH).

Published online:
17 June 2015Table 2. Formulation batches by the H69 process (precipitation + HPH).
In the CT method, the drugs were dispersed in 20 ml stabiliser solution and ball milled for 30 min at 400 rpm. Whereas in the H69 process, the organic solvent (1 ml methanol for ibuprofen and aprepitant) containing drug was added to the stabiliser solution and then was sonicated using a probe sonicator for 15 min at an amplitude of 60%. The prepared suspension was characterised using the Malvern zetasizer instrument for the particle size and polydyspersity index (PDI). The suspensions which showed particle size less than 1 µ were further subjected to HPH. In case of aprepitant, the suspension was homogenised for 5 cycles at 15,000 psi and the ibuprofen suspension was homogenised for 5 cycles at 10,000 psi.
Bcs Class 1 Drugs
2.2. Characterisation
The prepared nanosuspensions were evaluated for the particle size, PDI and zeta potential using Malvern zetasizer. The per cent yield was computed to measure the amount of drug present in the prepared nanosuspension. The nanosuspensions were lyophilised using mannitol as cryoprotectants for solubility studies, dissolution studies, Fourier transform infrared spectroscopy (FT-IR) and differential scanning calorimetry (DSC) analysis.
2.3. Optical microscopy
The pure drugs, aprepitant, ibuprofen and its dispersions of freeze dried products (ABH 4 and IBH 5) were observed under microscope, Motic BA400, to assess the size reduction qualitatively and images were captured.
2.4. Solubility studies
An excess amount of the freeze dried product, ABH 4 and pure drug, aprepitant were added separately to 5 ml each of distilled water and 2.2% w/v SLS solution. Similarly, excess amount of ibuprofen and nanoformulation, IBH 5 were added in 5ml of water, 0.1N HCl and phosphate buffer, pH 7.2. Then, the mixtures were mounted on the rotospin apparatus for 24 hours at room temperature at 50 rpm. After 24 hours, the solutions were filtered through a 0.22 μ membrane filter and the amount of drugs, ibuprofen and aprepitant, dissolved were determined spectrophotometrically at 220 and 210 nm, respectively.
2.5. Dissolution studies
The in vitro dissolution studies of both the pure drugs and its optimised nanoformulations were carried out using the USP II apparatus.[11 Dissolution methods [Internet]. Available from: http://www.accessdata.fda.gov/scripts/cder/dissolution/[Google Scholar]] The dissolution studies of aprepitant and its nanoformulation (freeze dried) were carried out in the medium, 2.2% SLS. Whereas, the dissolution of ibuprofen was carried out in 0.1N HCl and phosphate buffer, pH 7.2. The temperature of the dissolution medium was maintained at 37 ± 0.5 °C by a thermostatically controlled water bath. At predetermined time intervals of 10, 20, 30 and 45 min, 5 ml of sample was withdrawn, filtered and analysed spectrophotometrically at 210 nm in case of aprepitant. Whereas, in case of ibuprofen, aliquots were collected at the time intervals, 5, 10, 20, 30, 45 and 60 min and analysed spectrophotometrically at 220 nm. At each time point, 5 ml of fresh buffer was replaced into the dissolution flask to maintain the sink conditions. The drug dissolved or released from nanoformulation and pure drug was statistically analysed using student's t-test.
2.6. Fourier transform infrared spectroscopy (FT-IR) and differential scanning calorimetry (DSC)
The freeze dried nanoformulations and pure drugs were analysed for FT-IR spectroscopy and DSC to assess the crystallinity of the drug in the nanoformulations. The FT-IR spectra and DSC thermograms of the pure drugs and nanoformulations were compared and reviewed for any changes or interactions.
3. Results and discussion
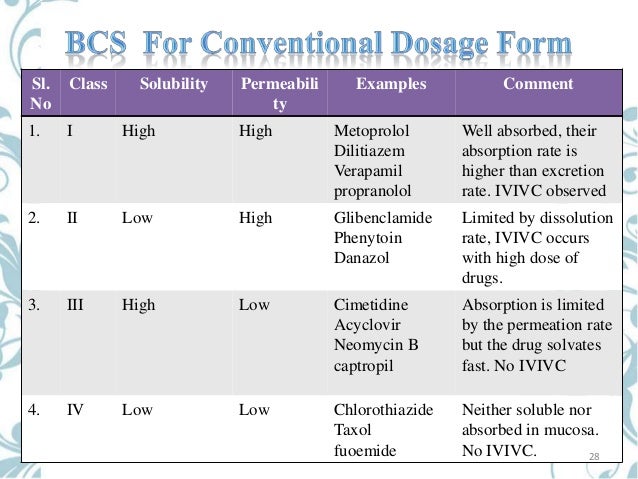
3.1. Formulation and characterisation of nanosuspensions
In the CT process, the aprepitant formulations (coded as ABH 1–5) were prepared by ball mill resulting in particles of size less than 1 µ were further subjected to HPH. HPH further reduced the particle size. The combination of tween 80 and poloxamer 188 (ABH 4) was observed to produce nanosuspension of 120.4 nm particle size with PDI of 0.268 and zeta potential of –31.26 mV (Figure 1). It has been observed that higher concentration of stabilisers than the drug, aprepitant, resulted in smaller particle size of nanosuspensions. In case of ibuprofen, formulations (IBH 7–10) failed to produce particles less than 1 µ and therefore, these samples were not further subjected to HPH. The suspensions stabilised with the polymeric stabilisers, such as PVA and poloxamer 188 did not fabricate particles of size less than 1 µ. However, poloxamer 407 (IBH 2) and tween 80 (IBH 5) suspensions produced particles of size 189.6 and 119.3 nm, respectively at the drug/stabiliser ratio 2:1. In contrary to aprepitant nanosuspension, lower concentrations of stabilisers resulted in smaller particle size of ibuprofen nanosuspensions. The percentage yield of aprepitant nanosuspension was found to be 90%, whereas it was 20% with 30 mg ibuprofen and 40%–50% in case of 20 mg ibuprofen.
Diablo 1 13 rune words. Download Diablo 2: Lord of Destruction Patch v1.13c. This patch updates Diablo 2: Lord of Destruction to version. This patch updates Diablo 2: Lord of Destruction to version. Diablo 2: Lord of Destruction Patch v1.13c. This patch updates Diablo 2: Lord of Destruction to version. Direct download. Patch 1.13c A new Mystery has been revealed! - Adventurers of Sanctuary are hereby warned once again, that a new challenge awaits you. Within Diablo's Bosses, spanning across the world from the ancient Monastery Catacombs to the Throne of Destruction.
Published online:
17 June 2015Figure 1. Effect of the CT process on the particle size, PDI and zeta potential of (a) aprepitant and (b) ibuprofen.
Figure 1. Effect of the CT process on the particle size, PDI and zeta potential of (a) aprepitant and (b) ibuprofen.
Aprepitant formulations, coded as APH 1–5 resulted in particles of size less than 1 µ after precipitation as pretreatment. Nevertheless, in the case of ibuprofen, the precipitation method as a pretreatment failed to produce particles less than 1 µ. The particle size obtained was in the range of 1 to 1.5 µ and therefore, these formulations were not further processed. HPH fabricated the particles of size 300–600 nm of the aprepitant nanosuspensions (Figure 2). The size of particles in the nanosuspension was observed to be in the lower range using lower concentrations of stabilisers, PVA and SLS, however, the stability of suspensions was not good enough. The use of tween 80 as a stabiliser resulted in a sticky mass. Combinations of stabilisers also failed to show any improvement in the size reduction or stability.
Published online:
17 June 2015Figure 2. Effect of the H69 process on the particle size, PDI and zeta potential of aprepitant.
Figure 2. Effect of the H69 process on the particle size, PDI and zeta potential of aprepitant.
It is observed from the above study that different process parameters and stabilisers are required to produce nanosuspensions of aprepitant and ibuprofen. The pressure of 10,000 psi of HPH was the most suitable in the case of ibuprofen, whereas aprepitant formulations were processed at 15,000 psi pressure. An increment in the pressure led to an increase in the particle size of ibuprofen nanosuspension. Two stabilisers, poloxamer 407 and tween 80, when used alone resulted in smaller particles of ibuprofen nanosuspension whereas the combination of poloxamer 188 and tween 80 produced smaller particles of aprepitant nanosuspensions. ABH 4 and IBH 5 were chosen as the optimised formulation depending on the particle size and further evaluated for other parameters. The formulations were lyophilised using mannitol (four times the amount of drug). The lyophilisation led to an increase in the particle size, however, it was still in the submicron range as indicated in Figure 3.
Published online:
17 June 2015Figure 3. Effect on the particle size after lyophilisation.
Figure 3. Effect on the particle size after lyophilisation.
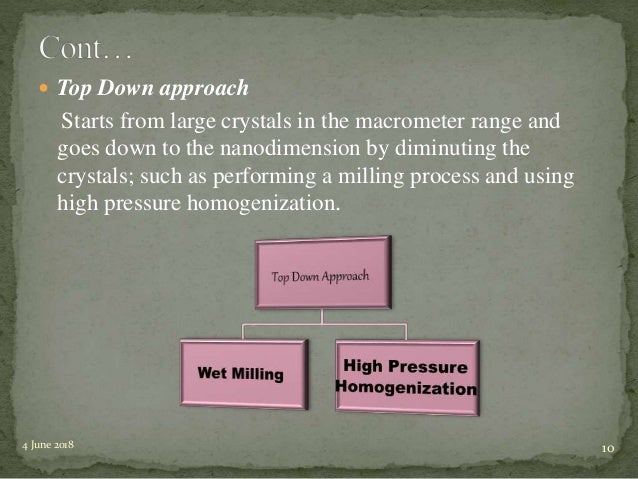
3.2. Optical microscopy
The particle size of nanoformulations was observed to be reduced qualitatively under microscope, as compared to pure drugs, which suggested the effective size reduction (Figure 4).
Published online:
17 June 2015Figure 4. Optical microscopy of aprepitant, ibuprofen and its nanoformulations.
Figure 4. Optical microscopy of aprepitant, ibuprofen and its nanoformulations.
3.3. Solubility studies
The nanoformulations of aprepitant and ibuprofen showed increased solubility than the pure drugs in all the vehicles used. The fold increase in the solubility is depicted in the Table 3. This showed that the reduction in the particle size led to increased surface area and thereby, increased solubility.
Published online:
17 June 2015Table 3. Solubility of aprepitant, ibuprofen and its optimized nanoformulations.
3.4. Dissolution studies
The release of aprepitant nanoformulation was found to increase significantly as compared to pure drug with 99% release in 45 min (Figure 5). Likewise, the release of ibuprofen nanoformulation was also found to increase as compared to the pure drug in the phosphate buffer, pH 7.2 with 95% release in 60 min. Although there was an increase in the dissolution profile in ibuprofen nanoformulation in 0.1N HCl than the pure drug, but the release achieved was 39% in 60 min (Figure 6). It has been observed that ibuprofen has a lesser dissolution profile in 0.1N HCl than the phosphate buffer, pH 7.2, which complies with the earlier report by Rivera-Leyva et al.[12Rivera-Leyva JC, Garcia-Flores M, Valladares-Mendez A, et al. Comparative studies on the dissolution profiles of oral ibuprofen suspension and commercial tablets using biopharmaceutical classification system criteria. Ind J Pharm Sci. 2012;74:312–318.[Crossref], [PubMed], [Web of Science ®], [Google Scholar]] The increase in the dissolution profile was observed to be statistically significant at p < 0.05 in both 0.1N HCl and phosphate buffer, pH 7.2.
Published online:
17 June 2015Figure 5. Dissolution profile of aprepitant and its nanoformulation.
Figure 5. Dissolution profile of aprepitant and its nanoformulation.
Published online:
17 June 2015Figure 6. Ipadian. Dissolution profile of ibuprofen and its nanoformulation in phosphate buffer, pH 7.2 and 0.1N HCl.
Figure 6. Dissolution profile of ibuprofen and its nanoformulation in phosphate buffer, pH 7.2 and 0.1N HCl.
3.5. FT-IR and DSC analysis
There was no considerable difference observed in the characteristic peaks with a slight shift in the values of the peaks in the FT-IR spectra of optimised nanoformulations of aprepitant and ibuprofen as compared to pure drugs (Table 4). The spectra of pure drugs, apreptitant, ibuprofen and its optimised nanoformulations are shown in the Figures 7 and 8, respectively. This indicated that there was no interaction between drugs and excipients. In DSC thermograms, the peak of ibuprofen in the nanoformulation was observed at 74 °C as compared to pure ibuprofen at 77 °C, which showed no major change in crystallinity of drug (Figure 9).[13Maheshwari M, Jahagirdar H, Paradkar A. Melt sonocrystallization of ibuprofen: Effect on crystal properties. Eur J Pharm Sci. 2005;25:41–48.[Crossref], [PubMed], [Web of Science ®], [Google Scholar]] However, a significant shift of melting peak of aprepitant in the nanoformulation was observed as compared to pure aprepitant (Figure 10).[14Ridhurkar DN, Ansari KA, Kumar D, et al. Inclusion complex of aprepitant with cyclodextrin: evaluation of physico-chemical and pharmacokinetic properties. Drug Dev Ind Pharm. 2013;39:1783–1792.[Taylor & Francis Online], [Web of Science ®], [Google Scholar]] This indicated the change in the crystalline nature of aprepitant during the preparation of nanosuspension. The shift also may be due to the higher concentration of stabilisers than the drug.[15Liu P, Rong X, Laru J, et al. Nanosuspensions of poorly soluble drugs: preparation and development by wet milling. Int J Pharm. 2011;411:215–222.[Crossref], [PubMed], [Web of Science ®], [Google Scholar]]
Published online:
17 June 2015Table 4. Characteristic peaks of aprepitant, ibuprofen and its optimised nanoformulations in FT-IR spectra.
Published online:
17 June 2015Figure 7. FT-IR spectra of (a) aprepitant and (b) its optimised nanoformulation.
Figure 7. FT-IR spectra of (a) aprepitant and (b) its optimised nanoformulation.
Published online:
17 June 2015Figure 8. FT-IR spectra of (a) ibuprofen and (b) its optimised nanoformulation.
Figure 8. FT-IR spectra of (a) ibuprofen and (b) its optimised nanoformulation.
Published online:
17 June 2015Figure 9. DSC thermograms of (a) aprepitant and (b) its optimised nanoformulation.
Figure 9. DSC thermograms of (a) aprepitant and (b) its optimised nanoformulation.
Published online:
17 June 2015Figure 10. DSC thermograms of (a) ibuprofen and (b) its optimised nanoformulation.
Figure 10. DSC thermograms of (a) ibuprofen and (b) its optimised nanoformulation.
4. Conclusion
Sinha et al. (2013)Sinha B, Muller RH, Moschwitzer JP. Systematic investigation of the cavi-precipitation process for the production of ibuprofen nanocrystals. Int J Pharm. 2013;458:315–323.[Crossref], [PubMed], [Web of Science ®], [Google Scholar] reported to produce particles of size 300 nm of ibuprofen by cavi-precipitation (combination of precipitation and HPH).[16Sinha B, Muller RH, Moschwitzer JP. Systematic investigation of the cavi-precipitation process for the production of ibuprofen nanocrystals. Int J Pharm. 2013;458:315–323.[Crossref], [PubMed], [Web of Science ®], [Google Scholar]] In this study, combination of ball milling and HPH produced particles of size near to 100 nm (though not less than 100 nm) of the drugs, aprepitant and ibuprofen. Combination of precipitation and HPH fabricated aprepitant particles of size 300 mm but it was not proved successful as a pretreatment process for ibuprofen in the present case. However, combination of two techniques, i.e., ball milling and HPH has reduced ball milling time (30 min in the present case) as well as the number of cycles to 5 in HPH to produce smaller particles. The optimised nanoformulations of ibuprofen and aprepitant also showed improved solubility and dissolution profile over pure drugs. This shows that the combination method is preferred over the conventional method in fabricating smaller particles with lesser processing time. However, the choice of methods depends on the nature of drugs and excipients used. Moreover, we are exploring other methods to produce particles of size less than 100 nm of aprepitant and ibuprofen and understand the effect of different combinational methods.
List of abbreviations
CT | combinative technologies |
HPH | pressure homogenisation |
Acknowledgements
The authors are thankful to Manipal University for providing the facilities and instruments to carry out this work.
Disclosure statement
No potential conflict of interest was reported by the authors.
Table 1. Formulation batches by the CT process (ball milling + HPH).
Code | Drug amount (mg/10 ml) | Polymer | Drug:polymer ratio |
---|---|---|---|
ABH 1 | Aprepitant 50 mg | Tween 80 | 1:2 |
ABH 2 | Aprepitant 50 mg | Poloxamer 188 | 1:6 |
ABH 3 | Aprepitant 50 mg | PVA | 2:1 |
ABH 4 | Aprepitant 50 mg | Tween 80 + poloxamer 188 | 1% tween 80 + 3% poloxamer 188 |
ABH 5 | Aprepitant 50 mg | PVA + poloxamer 188 | 0.25% PVA + 3% poloxamer 188 |
IBH 1 | Ibuprofen 30 mg | Poloxamer 407 | 2:1 |
IBH 2 | Ibuprofen 20 mg | Poloxamer 407 | 2:1 |
IBH 3 | Ibuprofen 20 mg | Poloxamer 407 | 4:1 |
IBH 4 | Ibuprofen 30 mg | Tween 80 | 3:1 |
IBH 5 | Ibuprofen 20 mg | Tween 80 | 2:1 |
IBH 6 | Ibuprofen 20 mg | Tween 80 | 4:1 |
IBH 7 | Ibuprofen 30 mg | Poloxamer 188 | 2:1 |
IBH 8 | Ibuprofen 20 mg | Poloxamer 188 | 2:1 |
IBH 9 | Ibuprofen 30 mg | PVA | 2:1 |
IBH 10 | Ibuprofen 20 mg | PVA | 2:1 |
Table 2. Formulation batches by the H69 process (precipitation + HPH).
Code | Drug amount (mg/10 ml) | Polymer | Drug:polymer ratio |
---|---|---|---|
APH 1 | Aprepitant 62.5 mg | PVA | 2.5:1 |
APH 2 | Aprepitant 62.5 mg | SLS | 1:6 |
APH 3 | Aprepitant 62.5 mg | Tween 80 + SLS | 1% tween 80 + 3% SLS |
APH 4 | Aprepitant 62.5 mg | PVA + SLS | 0.25% PVA + 3% SLS |
APH 5 | Aprepitant 62.5 mg | PVA + SLS | 1% PVA + 3% SLS |
APH 6 | Aprepitant 62.5 mg | PVP-K30 + SLS | 1% PVP-K30 + 3% SLS |
APH 7 | Aprepitant 62.5 mg | Tween 80 + PVP-K30 + SLS | 1% tween 80 + 1% PVP-K30 + 2% SLS |
APH 8 | Aprepitant 62.5 mg | Tween 80 + PVP-K30 + SLS | 1% tween 80 + 1% PVP-K30 + 3% SLS |
IPH 1 | Ibuprofen 30 mg | PVA | 2:1 |
IPH 2 | Ibuprofen 30 mg | PVA | 1:1 |
IPH 3 | Ibuprofen 30 mg | Poloxamer 407 | 2:1 |
IPH 4 | Ibuprofen 30 mg | Poloxamer 407 | 1:1 |
IPH 5 | Ibuprofen 30 mg | Tween 80 | 2:1 |
IPH 6 | Ibuprofen 30 mg | Tween 80 | 1:1 |
IPH 7 | Ibuprofen 30 mg | Poloxamer 188 | 2:1 |
IPH 8 | Ibuprofen 30 mg | Poloxamer 188 | 1:1 |
Table 3. Solubility of aprepitant, ibuprofen and its optimized nanoformulations.
Medium | Pure drug concentration (µg/ml) | Nanoformulation concentration (µg/ml) | Fold increase in solubility |
---|---|---|---|
Aprepitant | |||
Water | 16.67 | 671.30 | 40.269 |
2.2% SLS | 697.78 | 1428.52 | 2.047 |
Ibuprofen | |||
Water | 318.98 | 1054.88 | 3.307 |
0.1N HCl | 166.14 | 6443.72 | 38.784 |
Phosphate buffer, pH 7.2 | 1234.09 | 5085.81 | 4.121 |
Table 4. Characteristic peaks of aprepitant, ibuprofen and its optimised nanoformulations in FT-IR spectra.
Pure drug | |||
---|---|---|---|
Peaks | Reported (cm−1) | Observed (cm−1) | Nanoformulation (cm−1) |
Aprepitant | |||
C = O stretching | 1702 | 1703 | 1709 |
C = C stretching | 1600 to 1500 | 1507 | 1611 |
C–F stretching | 1400 to 1100 | 1175 | 1095 |
Ibuprofen | |||
C = O stretching | 1720 | 1718 | 1722 |
Bonded –OH stretching | 2955 | 2955 | 2934 |
- Muller RH, Gohla S, Keck CM. State of the art of nanocrystals – special features, production, nanotoxicology aspects and intracellular delivery. Eur J Pharm Biopharm. 2011;78:1–9., [Google Scholar]
- Junghanns J-UAH, Muller RH. Nanocrystal technology, drug delivery and clinical applications. Int J Nanomed. 2008;3:295–309., [Google Scholar]
- Merisko-Liversidge E, Liversidge GG. Nanosizing for oral and parenteral drug delivery: a perspective on formulating poorly-water soluble compounds using wet media milling technology. Adv Drug Deliv Rev. 2011;63:427–440., [Google Scholar]
- Keck C, Kobierski S, Mauludin R, et al. Second generation of drug nanocrystals for delivery of poorly soluble drugs: Smartcrystal technology. Dosis. 2008;24:124–128.
- Shegokar R, Muller RH. Nanocrystals: industrially feasible multifunctional formulation technology for poorly soluble actives. Int J Pharm. 2010;399:129–139., [Google Scholar]
- Chawla SP, Grunberg SM, Gralla RJ, et al. Establishing the dose of the oral NK1 antagonist aprepitant for the prevention of chemotherapy-induced nausea and vomiting. Cancer. 2003;97:2290–2300., [Google Scholar]
- Kockbek P, Baumgartner S, Kristl J. Preparation and evaluation of nanosuspensions for enhancing the dissolution of poorly soluble drugs. Int J Pharm. 2006;312:179–186., [Google Scholar]
- Moschwitzer JP, Lemke A. Gentle method for preparing ultrafine particle suspensions, useful for formulating e.g. pharmaceuticals, cosmetics, foods or pesticides, comprises freezing non-aqueous solution then dispersion of the frozen product. German patent application DE102005053462 A1. 2007 May 10.
- Kobierski S, Keck CM. Nanocrystal production by BMHPH combination technology. Controlled Release Society, Abstract, Germany Chapter, Annual Meeting, 2008 Mar 4–5, 40.
- Peterson R. Nanocrystals for use in topical cosmetic formulations and method of production thereof. U.S. patent 0,047,297 A1. 2010Feb 25.
- Dissolution methods [Internet]. Available from: http://www.accessdata.fda.gov/scripts/cder/dissolution/
- Rivera-Leyva JC, Garcia-Flores M, Valladares-Mendez A, et al. Comparative studies on the dissolution profiles of oral ibuprofen suspension and commercial tablets using biopharmaceutical classification system criteria. Ind J Pharm Sci. 2012;74:312–318., [Google Scholar]
- Maheshwari M, Jahagirdar H, Paradkar A. Melt sonocrystallization of ibuprofen: Effect on crystal properties. Eur J Pharm Sci. 2005;25:41–48., [Google Scholar]
- Ridhurkar DN, Ansari KA, Kumar D, et al. Inclusion complex of aprepitant with cyclodextrin: evaluation of physico-chemical and pharmacokinetic properties. Drug Dev Ind Pharm. 2013;39:1783–1792., [Google Scholar]
- Liu P, Rong X, Laru J, et al. Nanosuspensions of poorly soluble drugs: preparation and development by wet milling. Int J Pharm. 2011;411:215–222., [Google Scholar]
- Sinha B, Muller RH, Moschwitzer JP. Systematic investigation of the cavi-precipitation process for the production of ibuprofen nanocrystals. Int J Pharm. 2013;458:315–323., [Google Scholar]